⬤
◒
Lumbar Spine Biomechanics
From WikiMSK
This article is still missing information.
Movements
Movement | Description | Movement occurs when | Force |
---|---|---|---|
Translation | Every point on a bone moving in the same direction and to the same extent | Single force or net single force acts on a bone | Shear force |
Rotation | All the points on a bone move in parallel around a curved path that is centred on a fixed point, but to different extents depending on their distance from the centre of rotation. May be due to two opposed muscular actions, or muscular action and ligamentous resistance, or gravity opposed by either muscular action or ligamentous resistance. | Two unaligned forces acting in opposing directions on different parts of the bone forming a force couple | Torque |
- Axis of rotation
- Formal definition = that region that does not move when two or more opposing, unaligned forces act on a bone.
- With rotation of a bone, all the points on the bone can be grouped into individual planes that lie parallel to the direction of movement.
- In each plane, the points move about a centre located in that plane
- When all the centres of all the planes line up they depict a straight line forming the axis of rotation of the bone.
- It is a region where all opposing forces cancel out, and there is no net force acting, and so it stays stationary.
- Different forces create different axes of rotation.
Planes of Movement
Stress-Strain
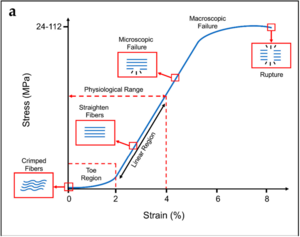
Typical stress–strain curve and schematization of the behaviour of the collagen fibres for tendons. Typical ranges of stress and strain are indicated on the x and y axes.[1]
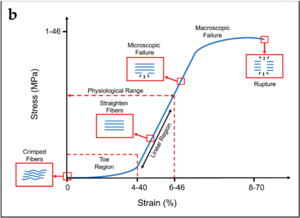
Typical stress–strain curve and schematization of the behaviour of the collagen fibres for ligaments.[1]
- See also: Fibrous Connective Tissues
With stretching of a collagen fibre, the fibre resists elongation due to a resistance force from the chemical bonds between the collagen fibrils, between tropocollagen molecules, between collagen fibres, and between collagen fibres and proteoglycans.
- Stress = the applied elongating force, measured in units of force (newtons)
- Strain = the extent to which a fibre is elongated, measured as the fractional or percentage increase in length relative to the initial length. A fibre of length L0 when stretched to a new length L1 undergoes a strain of L1/L0 x 100%
- Tension strain = this occurs with a deforming force causing a structure to be stretched longitudinally
- Compression strain = this occurs with a deforming force causing a structure to be squashed. Measured as the fractional or percentage decrease in height of a structure
- Shear force = forces that cause two vertebrae to slides with respect to one another
- Shear strain = the strain that occurs in the intervening intervertebral disc
- Shear vs tension: tension conventionally applies to forces that are exerted along the long axis of a structure. Shear forces are applied across the axis.
- Torsion = when an object twists, the force is a torque, and the resultant strain is a torsion strain.
- Crimp = At rest, single collagen fibres are usually buckled, the wavy shape is called crimp
- Failure = Collagen fibres have broken and cease to resist elongation
Stress-strain curve
- Three main regions
- First region is the toe phase. This is where the crimp is being removed from the collagen fibre with the application of stress, this requires little energy due to no major chemical bonds
- The second, linear region is the steep slope along the middle of the curve, and is where the stress stretches the collagen fibre longitudinally and the crimp has been removed. The bonds within and between the collagen fibrils and tropocollagen molecules are being strained and some are being broken. The peak is the phase of failure of the collagen fibre, with substantial numbers of bonds being irreversibly broken.
- The last part of the curve is once failure has occurred, and elongation occurs with ever decreasing amounts of stress Collagen fibrils start to be strained and broken somewhere after 3% and 4% of elongation of the fibre. About 4% is the maximum a fibre can sustain without risking microscopic damage.
- Collagenous tissues, like ligaments and joint capsules, behave similarly to isolated collagen fibres and show similar stress-strain curves, but with additional mechanical events
- In the toe phase, in addition to the removal of crimp, this phase may also represent the removal of any macroscopic slack in the ligament
- In the second phase, the collagen fibres are being re-arranged in the stress structure. Fibres that are curved or run obliquely at rest are straightened to line up with the applied force. Water and proteoglycans may be displaced. Bonds between separate collagen fibres and between collagen fibres and their surrounding proteglycan matrix are strained. This results in a steep slope of the second phase. After re-arrangement of the fibres and displacement of water, then the individual collagen fibres are strained.
- It isn't known what proportion of collagen fibres need to fail before macroscopic failure occurs, and cannot be predicted, therefore different structures need to be separately tested with several samples to produce an average stress-strain curve of a specific structure.
- A very important point is omitted from Bogduk's lumbar spine anatomy book. Tendons have specific load transfer functions, and the toe region of their stress-strain curve is short (2-5%), and is similar with each tendon in the body. Ligaments on the other hand allow different ranges of motion in different joints, and have wider ranges of strain for the toe region depending on the anatomical site (ACL is 4%, spine ligaments are 10-40%).[1]
- Clinical examination only evaluates no further than just beyond the toe phase.
Stiffness
- Stiffness = resistance to deformation
- Measured by the force required to product a unit elongation or deformation
- The slope of the stress-strain curve of a structure
- Stiffer structures resist deformation and have a steeper stress-strain curve
- It implies greater degree of bonding between collagen fibres, or between collagen fibres and their surrounding matrix
Initial Range of Movement
- This is where with movement of a joint, the resistance in the capsule and ligaments of the joint balances exactly the force attempting to move the joint.
- In a stress-strain curve, the initial range of movement is found somewhere early in the second phase of the curve just after the toe phase when collagen bonding is starting to resist movement
- With application of a greater force, the resisted structures and strained, and there is a new greater initial range of movement. This is dependent on the increase in force and the stiffness of the joint and ligaments
- To obtain a substantially greater initial range of movement, considerably larger forces are needed to most structures, and aren't usually possible during clinical examination.
- Even if the applied forces differs depending on the strength of the examiner, the resistance of the joint prevents great differences in perceived range of motion.
- The initial range of movement as evaluated in clinical examination falls in a narrow range and is called the normal range of movement.
Viscoelasticity
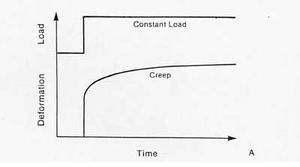
Creep. despite the maintenance of a constant load, elongation occurs with the passage of time.[2]
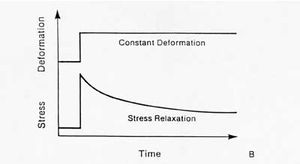
Stress relaxation. The stress is reduced or relaxed under a constant deformation[2]
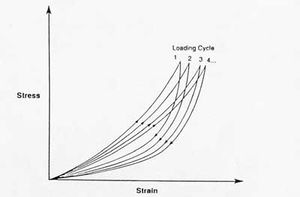
Hysteresis. During cyclic loading of a viscoelastic material, the stress-strain curve gradually shifts to the right.[2]
- Bogduk omits the explanation that ligaments/tendons have viscoelasticity.
- Viscoelasticity indicates time dependent mechanical behaviour.
- The relationship between stress and strain is not constant, but it depends on the time that a load is applied.
- There are three behaviour characteristics of viscoelasticity: creep, stress relaxation, and hysteresis.
Creep
- Creep is increasing deformation under constant load. This contrasts with an elastic material which does not exhibit an increase in deformation regardless of the length of time a load is applied.
- Initial ranges of movement are usually measured with brief application of force and applied until maximal range of motion, and then the force is released.
- However with a constant force over a prolonged period there is further movement that can be detected.
- This may be due to the gradual rearrangement of collagen fibres, proteoglycans, and water in the structure being stressed.
- Many occupations have regular prolonged load bearing of their lumbar spines in flexion, and their lumbar joints will creep.
Stress Relaxation
- Bogduk omits describing stress relaxation, an important behaviour of viscoelasticity.
- Stress relaxation means that the stress acting upon a tendon or ligament is eventually reduced under a constant deformation.
Hysteresis
- If a viscoelastic material is loaded and unloaded, the unloading curve will not follow the loading curve.
- Restoration of the initial length of a ligament occurs at a lesser rate and to a lesser extent than the deformation.
- The difference between the two curves represents the amount of energy that is dissipated or lost during loading.
- The energy used to displace water for example is no longer available, and if chemical bonds are broken they can't act to restore the form of the structure
- Set = the difference between the initial and final length.
- Hysteresis and a residual set don't occur with stress of a structure only in the toe phase, but the further a structure is stressed beyond this phase, the greater the hysteresis and set.
- Set disappears with time as collagen fibres and proteoglycans are rearranged into their standard configuration, and any displaced water is reabsorbed.
- If the original bonds are not reformed, or if new bonds are formed in the set position, then the set may persist indefinitely.
- A set often occurs following creep.
- Clinical applications
- In trauma, the energy lost in breaking the tissue may not be recoverable, and the original structure may not be fully reformed.
- Healing may occur in a set position, compromising the mechanical function of the structure.
- Healing in a set position effectively lengthens the ligament, and it will accommodate greater than normal initial ranges of movement, which may not be desirable, for example when an Achilles tendon heals in a long position.
- With sustained insults to ligaments and capsules, the ligament may be subject to forces within its load bearing capacity, but when a time factor is added, the ligament will creep. The ligament needs time to return to its usual configuration. Before this happens the structure may be liable to injury as it can't sustained reapplied loads in the usual way.
Fatigue Failure
Forces and Moments
Bibliography
- Bogduk, Nikolai. Clinical and radiological anatomy of the lumbar spine. Chapter 7. Edinburgh: Elsevier/Churchill Livingstone, 2012.
References
- ↑ 1.0 1.1 1.2 Sensini & Cristofolini. Biofabrication of Electrospun Scaffolds for the Regeneration of Tendons and Ligaments. Materials (Basel, Switzerland) 2018. 11:. PMID: 30322082. DOI. Full Text.
- ↑ 2.0 2.1 2.2 BME 332: Introduction to Biosolid Mechanics. Section 9: Ligament/Tendon Structure-Function. From http://umich.edu/~bme332/ch10ligten/bme332ligamenttendon.htm
Literature Review
- Reviews from the last 7 years: review articles, free review articles, systematic reviews, meta-analyses, NCBI Bookshelf
- Articles from all years: PubMed search, Google Scholar search.
- TRIP Database: clinical publications about evidence-based medicine.
- Other Wikis: Radiopaedia, Wikipedia Search, Wikipedia I Feel Lucky, Orthobullets,