Fibrous Connective Tissues
It could be said that Musculoskeletal Medicine is the study of disorders of fibrous connective tissues. Fibrous connective tissues are those tissues that are designed to withstand mechanical deformation.
Structural Overview
Deformation comes in two principal forms, tension and compression. However there are also shear forces. To withstand tension the body forms "strings" in the form of collagens. To withstand compression the body develops a fluid "mush" in the form of proteoglycans.
The fibrous connective tissues exist in a spectrum of composition. The more a tissue is required to withstand tension the more collagen it will have, while the more it is required to withstand compression the more proteoglycans it will have.
Tendons (principally collagen) -> ligaments (principally collagen plus 0.5% proteoglycans) -> fascia (mixed collagen and proteoglycans) -> cartilage (principally proteoglycans plus some collagen) -> bone (essentially cartilage that has been doped by calcium)
These various components are synthesised by cells. Fibroblasts manufacture the components of tensile tissues. Chondroblasts produce the components of cartilage. Osteoblasts form the components of bone. These various cells can transform into one another for example ligaments can become cartilaginous and then osseous.
Collagen in Tendons and Ligaments
Tendons
The primary functional unit of collagen is tropocollagen, which consists of a triple helix of polypeptide chains of around 1000 amino acid residues each. Tropocollagen measures around 3000 ร long by 15 ร wide.
Tropocollagen fibres aggregate end-to-end and side-to-side in a semi-overlapping pattern. The side-to-side tropocollagen fibres are joined by cross-links that occur during the normal maturation process. There is also end-to-end bonding.
One of the sources of resistance to tension is the end-to-end bonding between the polypeptide chains. Otherwise this triple helical structure when subjected to tension has the spaces between the helices taken up a little as it flattens out without being disrupted. However there is an electrical repulsion resisting this deformation.
Collagen fibres do have some spiralling, but they are principally organised longitudinally. Interspersed between the fibres are fibroblasts that synthesise the collagen.
Collagen is a very long-lived molecule with a slow rate of turnover. With normal ageing, there is an accumulation of cross-linking, which increases the stiffness and resistance to stretch.
Tendon Supporting Structures
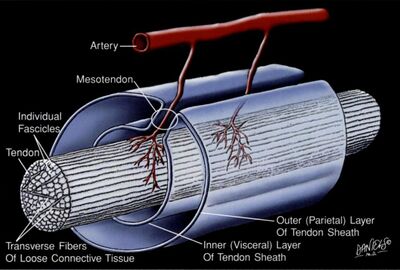
The individual fascicles are bound by other binding collagen that comes by various names. The little septae that go into the bundles of collagen are called endotenon. The surrounding structure is called epitenon. At the gross anatomy level, there is a loose surrounding fascial layer that surrounds some tendons called the paratenon. This is like the fascia of the tendon.
In locations of high friction the fibre is endowed by a layer of synovial membrane that secretes synovial fluid. This membrane wraps around the tendon in a way that is analogous to that of the abdomen. The internal layer is called the visceral tenon. There is an outer layer called the parietal tenon. The two layers are continuous and the site where the two layers develop is called the mesotenon. The lubricating layer is located between the parietal and visceral tenons. In areas with less friction, the tendon is surrounded by paratenon only. In the hands and toes the whole apparatus is further protected by fibrous capsules.
Inflammation of tendons is most commonly located in the surrounding tissue, not in the tendon itself. Disorders of the tendon are best referred to as tendinopathy, not tendinitis. Tenosynovitis is a term referring to inflammation of the surrounding synovial membrane layers. While paratendonitis refers to inflammation of the paratenon.
Ligaments
There is a continuum from the linear tendon structure to the planar ligament structure. There are fibres that run parallel to one another, endowed with fibroblasts that produce their constituents (mainly collagen). However within a ligament there are different layers, that can have slightly different orientations. Overall there is an average singular orientation, with some left or right deviation. This is because a ligament, unlike a tendon, doesn't sustain forces just in one direction.
Within a ligament itself, there is the same repetition of microstructure starting with tropocollagen with progressively increasing hierarchical organisation.
At rest the collagen fibres within the microscopic fibre are bent/coiled, i.e. in a state of crimp. The state of crimp in ligaments is important in the context of the clinical examination. This is because in normal clinical testing there is only a trivial amount of force produced, and so you are really only testing the amount of slack or crimp that the ligament has at rest. I.e. you are testing the toe phase of the stress-strain curve (see biomechanics below).
Irregular Fibrous Connective Tissue
- See also: Fascia
Irregular fibrous connective tissue is designed to resist forces in both tension and compression. Collagen fibres are arranged in different directions in order to resist tension in different directions. To resist compression the bundle of collagen fibres is endowed by proteoglycans.
The cardinal example of irregular fibrous connective tissue is subcutaneous fascia. This tissue has fat within it that supplements the anti-compression properties. Skin is able to stretched in different directions, and it is the collagen that resists this. Skin can also be squashed which is resisted by proteoglycans within the fascia.
Fibrous connective tissues respond to the forces applied to it. When subjected to tension the fibroblasts make collagen, when subjected to compression they make proteoglycans. This can be seen with wound healing. When an incision is made along the line of tension, the wound is healed with collagen which is the desired effect. However if the incision is made against the line of tension, the wound bed doesn't experience tension and so they don't get the stimulus to make collagen. Instead the wound makes proteoglycans which are manifested as keloids.
Proteoglycans
Structure
Proteoglycans are chains of glycosaminoglycans hanging from a core protein. Glycosaminoglycans are simply long chains of sugars, that are characterised by their repeating units. The repeating units are a pair of 6-carbon structures: a hexose (also called hexuronic acid) and a hexosamine. Hexosamines are amino sugar linked by glycosidic bonds. Different hexoses and hexosamines give rise to different glycosaminoglycans.
The glycosaminoglycans commonly found in fibrous connective tissues are chondroitin-6-sulphate, chondroitin-4-sulphate, keratan sulphate, dermatansulphate, and hyaluronic acid. Hyaluronic acid has a significantly larger molecular weight.
In hyaluronic acid there is a carboxyl group in each pair of repeating units, and this carboxyl group can ionise. Chondroitin-4-sulphate has a carboxyl group, and a sulphate group, which are both ionisable. Similarly in chondroitin-4-sulphate, there are two ionisable radical groups. In keratan sulphate, there is only one ionisable sulphate radical. Hyaluronic acid is unsulphated.
Glycosaminoglycan | Hexuronic acid | Hexosamine |
---|---|---|
Chondroitin 4 Sulphate | glucuronic Acid | N-acetylgalactosamine 4 sulphate |
Chondroitin 6 Sulphate | glucuronic Acid | N-acetylgalactosamine 6 sulphate |
Dermatan Sulfate | Iduronic Acid | N-acetylgalactosamine |
Keratan Sulfate | galactose | N-acetylglucosamine |
Heparan Sulfate | glucuronic Acid | N-acetylglucosamine |
Hyaluronic Acid | glucuronic Acid Unsulfated | N-acetyl-D-glucosamine Unsulfated |
Glycosaminoglycans come together to stick onto a core protein through covalent bonds. Each one of these structures is a proteoglycan, bearing chondroitin-sulphate and keratan sulphate as the cardinal glycosaminoglycans. Hyaluronic acid, another glycosaminoglycans, gets together with proteoglycans to form an aggregated proteoglycan. To stabilise the aggregation, a link protein binds the head end of the core protein to the hyaluronic acid. The proteoglycan aggregate is very large.
Water Binding Potential
There is a great electrostatic capacity in this structure. The negative charges in the sulphate and the carboxyl groups are attracted to the positively charged ends of water molecules. This is one of the two key ways that the aggregated proteoglycan can bind water.
The other way that it binds water is through thermodynamic principles. Any water molecules are spontaneously moving due to its thermodynamic properties. With a free body of water all the molecules exhibit similar activity. However in the presence of proteoglycans, the physical presence slows down the activity of water, preventing its egress. The aggregated proteoglycan exists not as a straight structure, but is highly coiled.
In ageing there is an increase in the ratio of keratan sulphate to chondroitin sulphate. Keratan sulphate as above only has one ionisation group, and so the proteoglycans have relatively less charge. Therefore with ageing, as the KS:CS ratio increases, the electrostatic water binding capacity is reduced.
Synthesis
The fibroblast is discussed here as an archetype, but the same principals apply for chondroblasts and osteoblasts.
Fibroblasts synthesis tropocollagen, which are made up of polypeptides that are formed in the organelle rough endoplasmic reticulum. Tropocollagen is secreted to the exterior through vesicles. The tropocollagen then spontaneously aggregates into microfibrils that then aggregate into increasingly thick collagen fibres.
Fibroblasts also synthesise proteoglycans. The protein components are produced by the rough endoplasmic reticulum, which are then sent into the Golgi apparatus. The Golgi apparatus is a packaging apparatus that adds carbohydrates to the proteins for export out of the cell.
Biomechanics
With stretching of a collagen fibre, the fibre resists elongation due to a resistance force from the chemical bonds between the collagen fibrils, between tropocollagen molecules, between collagen fibres, and between collagen fibres and proteoglycans.
The biomechanics of collagen can be understood through the stress-strain curve. First, two key definitions:
- Stress = the applied elongating force, measured in units of force (newtons)
- Strain = the extent to which a fibre is elongated, measured as the fractional or percentage increase in length relative to the initial length. A fibre of length L0 when stretched to a new length L1 undergoes a strain of L1/L0 x 100%
The stress-strain curve therefore represents the behaviour of a collagen fibre when it is subjected to a force (stress) that ventures to elongate the fibre (strain).
There are three regions of the stress-strain curve
- Toe Region: This is where the crimp is being removed from the collagen fibre with the application of stress. Crimp refers to the resting state of collagen fibres being buckled and wavy. Deformation here requires little energy due to no resistance from major chemical bonds, but not much deformation occurs at this point. The wavy fibres and transformed into straight fibres at the end of this region.
- Linear Region: The second, linear region is the steep slope along the middle of the curve. Here the stress stretches the collagen fibre longitudinally after the crimp has been removed. The bonds within and between the collagen fibrils and tropocollagen molecules are being strained and there is some micro-failure. A large amount of stress is needed for only a small amount of elongation.
- Macroscopic Failure: The last part of the curve is once macro-failure has occurred, with substantial numbers of bonds being irreversibly broken. Elongation occurs with ever decreasing amounts of stress. Collagen fibrils start to be strained and broken somewhere after 3% and 4% of elongation of the fibre. About 4% is the maximum a fibre can sustain without risking microscopic damage.
Tendons have specific load transfer functions, and the toe region of their stress-strain curve is short (2-5%), and is similar with each tendon in the body. Ligaments on the other hand allow different ranges of motion in different joints, and have wider ranges of strain for the toe region depending on the anatomical site (ACL is 4%, spine ligaments are 10-40%).[3]
The most important property of collagen is its tensile strength. Collagen buckles when it is compressed. In articular cartilage the tensile strength properties of collagen is complementary to proteoglycans which ensure compressive strength, elasticity, and the lifespan of articular cartilage (see Synovial Joints).
Attachment Sites
The musculoskeletal system as a whole has both hard and soft tissues. The interfaces between these tissues have specialised structures that produce gradients in order to reduce stress concentrations at the junction sites. The interfaces between tendons/ligaments and bone are called entheses, while the interfaces between tendons and muscles are called myotendinous junctions. Further discussion is available through the linked articles.
Resources
See Also
References
- โ Erickson SJ. High-resolution imaging of the musculoskeletal system. Radiology. 1997 Dec;205(3):593-618. doi: 10.1148/radiology.205.3.9393511. PMID: 9393511.
- โ Sodhi H, Panitch A. Glycosaminoglycans in Tissue Engineering: A Review. Biomolecules. 2020 Dec 29;11(1):29. doi: 10.3390/biom11010029. PMID: 33383795; PMCID: PMC7823287.
- โ 3.0 3.1 3.2 Sensini & Cristofolini. Biofabrication of Electrospun Scaffolds for the Regeneration of Tendons and Ligaments. Materials (Basel, Switzerland) 2018. 11:. PMID: 30322082. DOI. Full Text.