Vertebral Compression Fracture
The compressive force on the thoracolumbar spine is the force that acts along the long axis of the spine as arises from tension in the paraspinal muscles and gravity. The compressive force is resisted mainly by the anterior column (vertebral bodies and discs), plus a variable amount of around ~15% from the posterior column (zygapophysial joints). Narrowing of the intervertebral discs leads to an increase of force taken up by the posterior column. It can also lead to the inferior articular process tip to impinge on the caudal lamina. With severe reduction of disc height, up to 90% of the compressive force is resisted by the posterior column.
The endplate is the weakest part of the vertebral body of the lumbar spine. Initial damage from compressive forces usually occurs in the endplate or in the trabeculae that support the endplate. It is thought that the endplate needs to be thin to allow nutrient diffusion into the intervertebral disc. The superior endplate of the vertebral body is thinner and weaker than the inferior endplate and has less trabecular support from the pedicles in the parasagittal plane. Please note that the superior endplate of the vertebral body is the same thing as the inferior endplate of the intervertebral disc. Failure occurs with the endplate bulging into the vertebra due to pressure from the nucleus pulposus.[1]
Normal and Young Vertebra
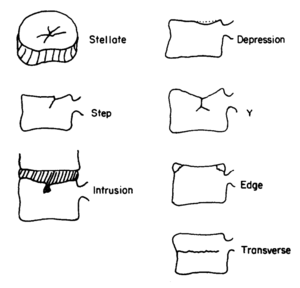
Various types of macroscopic endplate fracture have been found to occur in the lumbar spine (figure 1). In some instances there can be intrusion of disc material into the trabecular bone called intraosseous herniation. This defect can become calcified in what is called a Schmorl's node. In adolescents, endplate failure may occur differently with fracture of the posterior edge that runs from the endplate down to the cartilage growth plate. Endplate fractures are not always seen on plain films. MRI is more accurate and this modality can also show Modic changes which are reactions to the endplate fracture.
Burst fractures are comminuted vertebral body fractures where the anterior and posterior walls of the vertebral body are disrupted. They are characterised by an increased interpedicular distance. Bone can retropulse into the spinal canal causing neurological signs and symptoms. The adjacent intervertebral discs are also damaged with this injury and disc material is forced into the vertebral bodies. They are more common in the thoracic spine but resemble the transverse fracture type in figure 1. They are less likely to occur with intervertebral disc degeneration.[1]
At the microscopic level, it is thought that compressive fatigue damage is a common event throughout life because microfractures and healing trabeculae are found in most cadavers.[3]
Osteoporotic and Elderly Vertebra
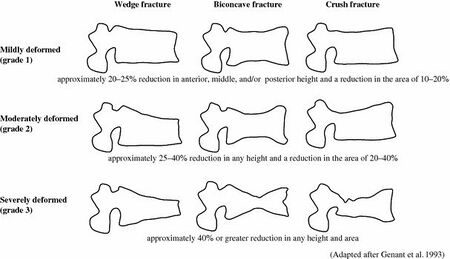
Osteoporotic fractures are fragility or low-trauma fractures. Vertebral compression fractures are the most common type of osteoporotic fracture. They often occur at the midthoracic region (T7-8) and at the thoracolumbar junction (T12-L1). They can result in significant back pain and loss of function however those that occur slowly over time are often asymptomatic. They also lead to height loss and kyphosis.
Types
Osteoporotic vertebra display different patterns of failure: anterior wedge fractures, biconcave fractures, and crush fractures (figure 2).[1]
Anterior wedge fractures are where there is collapse of the cortex of the vertebral body plus collapse of the underlying trabecular bone leading to a wedge shape. It is thought that with osteoporosis there is relative loss of thickness of the anterior trabeculae compared to the posterior trabeculae. There is also relative loss of the horizontal compared to the vertebral trabeculae, which reduces resistance to tension. Furthermore, the endplate is weaker anteriorly than posteriorly.
With intervertebral height reduction, more of the compressive load is taken up by the posterior column, and the anterior column is "stress-shielded" in the neutral and extended positions. However in the flexed position the anterior vertebral body is severely loaded in the degenerative disc. The elderly may spend less time in flexion leading to a reduced mechanical stimulus for hypertrophic adaptation. This combined with a reduced ability for the endplate to adapt (e.g. due to menopause), may explain why fractures occur in the anterior vertebral body and why flexion movements often precipitate it.[5]
Posterior wedging is uncommon and may suggest an underlying destructive lesion.
Biconcave fractures are also common in the elderly. In this type of fracture both central aspects of the endplates have collapsed inwards which are weaker than the outer aspects of the endplates. The endplates are often smooth which suggests repeated microfractures having occurred. In younger spines compression failure usually leads to more discontinuous endplate damage.
Back to the elderly, the discs may not have an overall reduced height even though degeneration has occurred due to the nucleus pushing into the weakened vertebra. The annulus fibrosus may be reduced in thickness, however, and this may be a better sign of intervertebral disc integrity than overall height or nucleus height.
Crush fractures are thought to be due to a sudden compressive force acting on the anterior and posterior vertebral cortices at the same time. In younger spines this type of trauma can cause burst fractures which have a similar appearance.
Grading
Osteoporotic spinal fractures are graded with the Genant classification based on vertebral height loss on lateral radiographs (figure 2):[6]
- Grade 0: normal
- Grade1 : mild, up to 20-25% reduction in anterior, middle, and/or posterior height
- Grade 2: moderate, up to 25-40% reduction in any height
- Grade 3: severe, >40% reduction in any height
Additionally, a modifier of 0.5 can be added for borderline deformed vertebrae.
Schmorl's Nodes and Modic Changes
As described, endplate fracture can lead to vertical herniation of the nucleus pulposus into the vertebral body. Calcification around this herniated tissue are called Schmorl's nodes when see on radiographs. Approximately 1/3 of endplate fractures as seen on MRI can be seen on plain radiographs. Yet smaller vertical herniations are seen in approximately 3/4 of spines in dissection.
Schmorl's nodes are most common near the thoracolumbar junction. They are rare below L2. They most commonly affect the central part of the endplate, and are more common on the inferior vertebral endplate than the superior endplate. Remember it is actually the superior endplate that is stronger, so one thought is that the endplates haven't formed properly in some people. Genetics play an important role with a heritability of 70%. There are also mechanical risk factors, namely: increased height, increased weight, and male gender. The prevalence increases with age up to 30, and then plateaus. Schmorl's nodes are also associated with disc degeneration. They are twice as common in patients with back pain, and are common in young sportsmen. (See also Scheuermann's Disease).
Modic changes are endplate patterns seen on MRI. Modic type 1 change is decreased signal intensity on T1, and increased signal intensity on T2, and reflects inflammatory change. Modic type 2 change is increased signal intensity on both T1 and T2 (or isointense on T2), and reflects fatty change. Type 1 can convert to type 2 after a few years. Inflammatory changes are thought to be more closely associated with pain than endplate injury itself. Modic changes are associated with confirmed discogenic pain by discography.[1]
Internal Disc Disruption
- Main article: Internal Disc Disruption
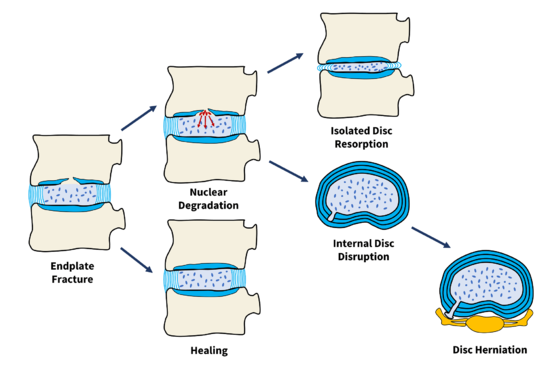
Endplate fractures can heal or it can trigger events leading to nuclear degradation (figure 3). Nuclear degradation in turn can lead to isolated disc resorption or internal disc disruption, the latter being an important cause of chronic low back pain. Internal disc disruption is the presence of isolated, radial fissures that penetrate from the nucleus pulposus through to the anulus fibrosus, however the outer anulus is not breached. It is a common
Endplate fracture is an important initial event in the pathogenesis of internal disc disruption. With compressive damage to the vertebra, the endplate bulges into the vertebral body. The volume of the nucleus pulposus increases, and therefore the pressure within the nucleus drops. This reduced pressure means it is less able to resist the applied compression loads, and so more of the load is placed on the annulus fibrosus, with peak loads on the posterior annulus. Inner lamellae can even collapse inwards.[1]
With endplate fracture, disc cell metabolism becomes impaired with very high and very low matrix compressive stresses. This leads to reduced proteoglycan synthesis in the nucleus, which further reduces the nucleus volume and pressure, and a vicious cycle emerges. With very high loads degradative pathways can also be stimulated.[1]
Young spines are better able to adapt because the inner annulus is more fluid-like and can accommodate the altered shape of the endplates better and so the pressure within the nucleus doesn't fall, and therefore there is less or no change in the stress on the annulus.[1]
At the other end of the spectrum of the age, old and severely degenerative discs may also be less affected by endplate damage. In this case the reason is due to stress shielding where the posterior column takes up more of the load. Any decompression of the nucleus therefore leads to extra compressive loading on the posterior column rather than the anulus.[7]
References
- โ 1.0 1.1 1.2 1.3 1.4 1.5 1.6 Adams et al. Mechanical damage to the thoracolumbar spine In: The biomechanics of back pain. Third edition. 2013
- โ Brinckmann P, Biggemann M, Hilweg D. Prediction of the compressive strength of human lumbar vertebrae. Clin Biomech (Bristol, Avon). 1989;4 Suppl 2:iii-27. doi: 10.1016/0268-0033(89)90071-5. PMID: 23906213.
- โ Vernon-Roberts B, Pirie CJ. Healing trabecular microfractures in the bodies of lumbar vertebrae. Ann Rheum Dis. 1973 Sep;32(5):406-12. doi: 10.1136/ard.32.5.406. PMID: 4270883; PMCID: PMC1006135.
- โ Brinckmann P, Biggemann M, Hilweg D. Prediction of the compressive strength of human lumbar vertebrae. Clin Biomech (Bristol, Avon). 1989;4 Suppl 2:iii-27. doi: 10.1016/0268-0033(89)90071-5. PMID: 23906213.
- โ Pollintine P, Dolan P, Tobias JH, Adams MA. Intervertebral disc degeneration can lead to "stress-shielding" of the anterior vertebral body: a cause of osteoporotic vertebral fracture? Spine (Phila Pa 1976). 2004 Apr 1;29(7):774-82. doi: 10.1097/01.brs.0000119401.23006.d2. PMID: 15087801.
- โ Genant HK, Wu CY, van Kuijk C, Nevitt MC. Vertebral fracture assessment using a semiquantitative technique. J Bone Miner Res. 1993 Sep;8(9):1137-48. doi: 10.1002/jbmr.5650080915. PMID: 8237484.
- โ Pollintine P, Przybyla AS, Dolan P, Adams MA. Neural arch load-bearing in old and degenerated spines. J Biomech. 2004 Feb;37(2):197-204. doi: 10.1016/s0021-9290(03)00308-7. PMID: 14706322.