Nerve Conduction Studies
Nerve conduction studies (NCS) allow the stimulation and recording of peripheral nerve function. Electrical stimulation causes an impulse that travels along motor, sensory, or mixed nerves. Potentials are recorded from either the muscle innervated by the motor nerve, or from the nerve itself. NCS is used to diagnose focal and generalised peripheral nerve disorders; aid in the differentiation between primary muscle and nerve disorders; classify peripheral nerve conduction abnormalities as being due to demyelination, axonal degeneration, or conduction block; and provide a prognosis on treatment effect and clinical course. [1][2]
Anatomy and Normal Neurophysiology
Most peripheral nerves are mixed, comprising motor, sensory, and autonomic functions. There are certain primarily motor (e.g. anterior interosseous branch of the median nerve, posterior interosseous branch of the radial nerve) and primarily sensory fibres (e.g. sural nerve, peripheral peroneal nerve, superficial radial nerves) that allow neurophysiologic investigation.
Individual nerves are generally classified based on their diameters, conductivity, and myelin properties. See table below. The largest Aα muscle afferent fibers are sometimes classified as 1a fibers.
- Characteristics of peripheral nerve fibres
Nerve Fibre | Myelin | Diameter (µm) | Conduction velocity (m/s) | General Function |
---|---|---|---|---|
Aα (I) | Yes | 13-20 | 80-120 | Proprioception: muscle spindle primary endings (Ia), golgi tendon organs (Ib), and alpha motor neurons |
Aβ (II) | Yes | 6-12 | 35-75 | Discriminative sensitivity to mechanical stimuli (touch, vibration), proprioception, pain modulation (block nociceptive information, allodynia in sensitisation) |
Aγ | Yes | 4-8 | 15-40 | Touch, pressure, and gamma motor neurons. |
Aδ (III) | Thin | 1-5 | 5-30 | "rapid" pain, crude touch, pressure, temperature. AMH type I for rapid mechanical pain (high heat threshold >53C), AMH type II for rapid heat pain (lower heat threshold 43-47C). |
B | No | 1-3 | 3-14 | preganglionic autonomic |
C (IV) | No | 0.2-1.5 | 0.5-2.0 | "second" pain, mechanical, chemical, thermal, pruritis, and postganglionic autonomic. polymodal |
Most efferent motor fibres are Aα (large myelinated). Aα fibres also include sensory fibres providing touch, vibration, and position senses. Cold and fast pain sensations are mediated by Aδ fibres (small myelinated). Warm, itch, and slow pain sensations are mediated by C fibres (unmyelinated). C fibres also include efferent postganglionic sympathetic autonomic fibres.[2]
Small fibre neuropathy - where strength, vibration, and position are normal, but pain and temperature are abnormal - poses a problem with nerve conduction studies. This is because NCS can only evaluate the largest A-alpha fibres. In other words, NCS is normal in pure small-fibre neuropathies. A skin biopsy may be required for diagnosis in this case.[2]
Compound action potentials
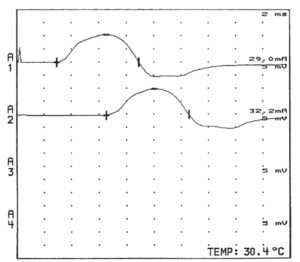
A-alpha fibres generate sensory nerve action potentials (SNAPs) and compound muscle action potentials (CMAPs). SNAPs and CMAPs are compound potentials representing summated electrical activity of individual nerve fibres with simultaneous activation of nerve stimulation. A-delta fibre electrical activity can be visualised through special techniques.[2]
Myelinated fibre nerve conductivity is saltatory, via Ranvier (node-to-node) depolarisation. The speed of conduction is dependent on the nerve fibre diameter, with the largest fibres conducting the fastest. The relationship between diameter and velocity is roughly linear at a conversion factor of around 4.3m/s/micron in healthy individuals. 14 to 15 micron A-alpha fibres have a conduction velocity of around 65 to 70 m/s, and 6 to 7 micron A-alpha fibres at around 30 to 35 m/s.[2]
The SNAP peak-to-peak amplitude and area under the waveform represents the number of activated nerve fibres. The CMAP amplitude relates more to the number of activated muscle fibres rather than nerve fibres.[2]
With an acute nerve lesion, before the onset of nerve regeneration, there is a loss of motor nerve fibres in parallel with the loss of activatable muscle fibres. Therefore CMAP amplitude is reduced. With chronic lesions, surviving motor units reinnervate the previously denervated muscle fibres through sprouting of collateral terminal motor nerve fibre branches. There are subsequently reduced numbers of motor fibres, each having increased motor unit territories and increased muscle fibre per motor units numbers. Therefore in this situation, CMAP amplitude is a poor estimate of motor nerve fibre number. The CMAP can have a normal or only mildly reduced amplitude. Motor unit number estimation (MUNE) and electromyography can be helpful in this situation.[2]
Temporal dispersion

The largest and fastest conducting A-alpha fibres contribute to the earliest part of the compound evoked potentials, while slower conducting fibres contribute to the later parts. This is called temporal dispersion of electrical activities. The onset latency of a compound evoked potential is determined by the conduction speed of the fastest fibres. The duration of the evoked potential can be measured from the time it leaves the baseline to the time when it returns to the baseline. [2]
An analogy for temporal dispersion is a race between a Greyhound and a Dachshund over a distance of 1 kilometre. The greyhound can run at a speed of 70km/hr, while the Dachshund can run at 30km/hr. Shortly after the start of the race, the dogs are bunched together. The dog "concentration" is at it's highest level, and this represents the CMAP amplitude. Also at the start, the spread between the Greyhound and Dachshund is shortest. As the race progresses, the dogs spread out, and so their concentration at any one point decreases. When the Greyhound reaches the finish line, the Dachshund is 1 minute and 10 seconds behind. At the finish line the concentration (amplitude) of dogs is less than previous points in the race, and the distance (duration) between the Greyhound and Dachshund is the greatest. In other words, with nerve conduction, the amplitude is greatest at the beginning, lessening as time goes on. This reflects the differences in conduction speed of the different A-alpha fibres. So when the distance increases between the stimulating and recording electrodes, you get an increase in temporal dispersion, and a decrease in amplitude.[2]
Temporal dispersion can be characterised as abnormal with an an excessive difference in slowing between individual motor axons within a nerve. This is generally due to altered or damaged myelin. There are different criteria defining abnormal temporal dispersion. For example the American Association of Neuromuscular and Electrodiagnostic Medicine define abnormal temporal dispersion as a 30 percent or more increase in CMAP duration between proximal and distal sites. Note that the relationship between increasing nerve length segment and temporal dispersion is roughly linear for motor conduction, but not for sensory conduction. [2]
Phase Cancellation
The recorded potentials of both single motor units and single sensory fibres have both positive and negative components. Therefore phase cancellation can occur depending on the conduction velocity of the individual fibres. Phase cancellation is more significant with increasing distances between the stimulating and recording electrodes. [2]
Individual sensory nerve fibres contribute differently to SNAPs, and individual motor nerve fibres contribute differently to CMAPs. A single motor unit potential has a much longer action potential than a single large myelinated sensory nerve fibre (5-15msec vs 2msec). With the shorter sensory nerve fibre potential, small amounts of dispersion can cause a dramatic reduction in SNAP amplitude compared to CMAP amplitude. Increasing the conduction distances decreases median and ulnar SNAP amplitudes by 16-17 times more than the corresponding CMAP amplitude decrease. The extent of SNAP amplitude reduction with increasing distance is not defined by any criteria. The distance is important when considering the diagnostic criteria for demyelinating nerve diseases.[2]
Saltatory Conduction
In myelinated fibres, nodes of Ranvier which are interspersed between myelinated internodal areas along the axon. Saltatory conduction refers to the rapid propagation of action potentials along myelinated fibres where conduction jumps from node to node along the fibre. There is activation of inward sodium currents, which generates an outward driving current at the next node. The membrane at the node is depolarised to a threshold, which opens sodium channels and initiates another cycle. Conduction occurs when the driving current exceeds the threshold current. The safety factor is the ratio of driving current to threshold current, normally over 5. Saltatory conduction allows conduction velocities of myelinated fibres to be over 10 times faster than predicted for unmyelinated axons of the same diameter.[2]
Nerve Conduction Physiologic Factors
- Different nerves and segments
- Specific nerves and segments vary in their distal latencies, conduction velocities, and evoked potentials. Upper extremity nerves conduct 15 to 20 percent faster than lower extremity nerves. Proximal segments conduct 5 to 10 percent faster than distal segments. Sensory conduction is 5 to 10 percent faster than motor conduction for each mixed nerve segment.[2]
- Age
- Full-term infants have nerve conduction velocities that are around half of that of adults. Velocity increases to the adult range by age 3-5. Velocities plateau until ages 25-30, and then decline slowly around 1-3 percent each decade. The loss of nerve fibres with age is thought to cause a reduction in evoked potential amplitude, but this varies from nerve to nerve, and is more pronounced with SNAP than CMAP. The SNAP in median, ulnar, sural, and superficial peroneal nerves can decline as much as 50-75% between the second and ninth decades. Loss of evoked potentials with surface electrode recording in normal older adults is common. [2]
- Body morphometry and gender
- Taller people have slower nerve conduction velocities, and this is more pronounced with lower extremity nerves. Women have faster conduction velocities than men, but this may be due to height differences. Differences in subcutaneous tissue may also be a factor with higher sural SNAP amplitudes in women at the ankle. Increased BMI may relate to decreases in SNAP amplitudes. Conduction velocity changes with increasing BMI can vary depending on the nerve segment being measured. Ethnicity can also effect normative values.[2]
- Temperature
- Decreases in local temperature results in increased evoked potential amplitudes, increased distal latencies, and decreased conduction velocities. Therefore standardisation of temperature during testing is vital. The arm should be maintained at 34 to 36°C and leg at 32 to 34°C during assessment. Two important factors to consider are anxiety-induced sympathetic overactivity and cold weather. External heating can alter measured skin temperature but may not alter nerve temperature. It is not recommended to use correction factors to compensate for low limb temperatures.[2]
Clinical Applications
In general, nerve conduction studies are used to assess diffuse processes such as polyneuropathies and motor neuron disease, as well as multi-focal processes such as mononeuritis multiplex and focal neuropathies such as carpal tunnel syndrome. They can allow precise anatomical localisation of lesions in the peripheral nervous system. They are used for classification of the underlying pathophysiology. They highly sensitive but in general the specificity is poor. Nerve conduction studies can help narrow down the pathophysiology (e.g. axonal vs demyelinating) and can give an idea as to the severity of the process. However, they are an adjunct to other more specific tests when a final diagnosis is made. A single study also only gives a snapshot in time, and so it cannot give an idea of the rate of progression of if the process is static.[1]
NCS are uncomfortable due to electrical stimulation, and it is vital to forewarn patients, and not lie to children that they don't hurt. Patient cooperation is essential due to the tiny responses that are recorded. Anything between the electrode and nerve can affect results such as oedema, very thick skin, or excess adipose tissue. Responses can be very difficult to record or even impossible in some instances. If the problem is reversible such as oedema, then it is best to delay the test until that is treated. [1]
NCS are very safe. There is a small theoretical risk with performing NCS in those with implanted defibrillators, especially when applying high frequency stimuli, because the implant could interpret it as ventricular tachycardia. Therefore it is sometimes recommended that defibrillators are turned off during the test by a cardiac technologist. Pacemakers are generally safe.[1]
In most situations, it is very important to perform NCS alongside electromyography (EMG). This is especially important in patients with suspected radiculopathy, plexopathy, myopathy, motor neuropathy, or motor neuron disease.[2]
Methodology
Three types of nerves can be tested: motor, sensory, and mixed. Motor nerves can be tested from the cell body to the muscle. Sensory nerves can only be tested distal to the dorsal root ganglion due to the unusual cell body anatomy. This discrepancy can be useful in localising the site of a lesion. Motor and sensory fibres are tested together with mixed nerves. Mixed nerves are generally the more proximal segments of the nerve, before the motor and sensory components have split.[1]
The essential parameters evaluated during clinical peripheral NCS are the amplitudes, areas, configurations, and durations of the sensory nerve action potentials (SNAPs) and compound muscle action potentials (CMAPs) evoked by peripheral nerve stimulation, and the sensory and motor nerve conduction velocities. All measures are dependent upon the integrity of the largest myelinated fibers. [1]
Sensory Studies
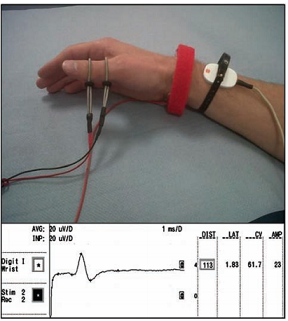
The superficial radial nerve is a commonly studied nerve. This nerve can be recorded in either direction. Orthodromic means in the normal direction (distal to proximal for sensory nerves), and antidromic is the opposite. The digital sensory branches of the radial nerve are stimulated using ring electrodes on the thumb. The recording electrode is placed over the radial nerve at the wrist. The inside of a nerve fibre is at a negative potential compared to the extracellular space. A cathode (negative, black) wire is used to apply a negative potential to the skin. This depolarises the nerve cell membrane and triggers an action potential. The stimulus is increased until the response does not get any bigger in order to stimulate all the fibres in the nerve. The stimulus is then increased by a further 10% to be confident that all nerve fibres are stimulated, called supra-maximal stimulation. It is important not to increase it further than this, because it is more uncomfortable and stimulation can spread to unwanted places affecting the result. The response is called SNAP (sensory nerve action potential). The SNAP is the sum of all the action potentials at the recording electrode. Only the large, myelinated fibres are recorded. Small unmyelinated fibre responses are variable and so they cannot be distinguished from the baseline.[1]
- Amplitude
Sensory responses amplitudes are very small, generally around 10µV. Therefore the neurophysiologist needs to average several responses to remove background nerve. It is very important that the patient is completely relaxed and that there is no interference from other electrical equipment. Difference in amplitudes between two sides, or between adjacent nerves, can often give more information than the absolute values. The fact that SNAPs are difficult to record means that if you see a normal SNAP then this is a relatively reassuring result on its on that there is no significant pathology in the peripheral nerve or plexus. In sensory axonal neuropathy ("dying back"), the amplitude reduces without much reduction in the conduction velocity, and distal nerve segments show a greater reduction in amplitude than proximal segments. Dorsal root ganglionopathies will generally give a comparable reduction in amplitude in the proximal and distal segments.[1]
- Conduction velocity
Conduction velocity is calculated by measuring the time (onset latency) between the stimulus and onset of the response. In the example shown of radial nerve stimulation, the latency is 1.83ms, the distance is 113mm, and so the conduction velocity is 61.7 m/s. The onset of the peak is measured, and so this represents the conduction velocity of the fastest fibres. Normal upper limb conduction velocities should be over 50 m/s, and lower limbs over 40 m/s.[1]
Conduction velocity is important when differentiating between axonal and demyelinating neuropathies. Axonal neuropathies show a drop in conduction velocity, but it never less than 70% of the normal value. Values below this suggest a demyelinating process. Conduction velocity is also important when localising the site of nerve compression. The focal demyelination in a compression site causes a drop in conduction velocity in that segment. In carpal tunnel syndrome, the first change seen is a slowing of median nerve sensory fibres at the wrist. This is often seen before any reduction in amplitude or alteration or motor conduction study results.[1]
Motor Studies
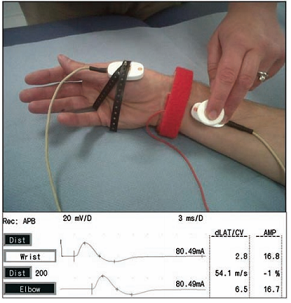
In motor studies a motor nerve is stimulated and the response is recorded over the muscle the nerve supplies. For example when measuring the median nerve, the nerve is stimulated at the wrist, and the response is recorded over the abductor pollicis brevis. The compound muscle action potential (CMAP) is the resulting potential. The CMAP is the sum of all the action potentials in the muscle fibres. A single motor nerve fibre supplies many muscles, and so the CMAP is an order of magnitude larger than a SNAP. Therefore it is easier to measure.[1]
- Conduction velocity
It is more complicated calculating the conduction velocity of a motor nerve than a sensory nerve. With CMAPs, the time between the stimulus and response includes the time for the action potential to travel along the nerve, the time taken for neuromuscular transmission, and the time for an action potential to travel along the muscle fibre. Neuromuscular transmission and muscle fibre transmission cannot be measured (known unknowns). Therefore you can't simply calculate the conduction velocity by simply using distance and time. Distal motor latency is referred to rather than velocity of the most distal portion of the nerve. Recording is done at a standard distance, and so it can be compared to normative values. For proximal segments (proximal latency), it is assumed that the unmeasurable factors are relatively constant within a few microseconds, and so they cancel out for these segments. Conduction velocity can therefore be calculated by taking the distance, and dividing by the difference between the distal latency and the proximal latency.[1]
Motor conduction velocity = d / (proximal latency - distal latency)
Velocity is more useful than latency because it is easier to compare nerves in a patient, and between patients. This is because the length of the tested segment is no longer relevant.[1]
- Amplitude
Absolute CMAP amplitude is less helpful with motor than sensory nerves. This is because the nerve is stimulated, but the muscle is recorded, and so the response reflects the activity of both of these. Collateral re-innervation can mean that you can lose a large amount of motor nerve fibres before you see a drop in CMAP amplitude. A reduced CMAP amplitude can mean a loss of motor nerve fibres, failed action potential propagation, failed neuromuscular junction transmission, or primary muscle disease. Repetitive nerve stimulation and electromyography can usually easily differentiate between these possibilities. Just as with sensory studies, any difference in amplitude between segments of the same nerve or between adjacent nerves is more useful information than any absolute value.[1]
- Temporal Dispersion and Conduction Block
Large myelinated fibres (what is recorded in NCS) all conduct at roughly the same velocity. Therefore the potential is a smooth peak. In an acquired demyelinated process (e.g. chronic inflammatory demyelinating polyneuropathy), there is a reduced conduction velocity in some of the fibres, and the reduction is variable. In this case, the CMAP is spread out rather than being a smooth peak - i.e. temporal dispersion. At first, the area under the curve is maintained meaning that all fibres still conduct but with reduced velocities. At some point conduction fails in a proportion of the fibres, and so the amplitude and area under the curve reduces. This is called conduction block. Conduction block can not simply be demonstrated by a reduced CMAP amplitude, because this can be caused by axonal loss. Conduction block is identified by a significant reduction of CMAP amplitude between distal and proximal stimulation sites within the same nerve. Not achieving supra-maximal stimulation can cause a drop in CMAP amplitude between a distal and proximal segment. Nerves tend to be deeper in more proximal sites, and it can sometimes be difficult to fully stimulate them. Some examples are stimulating the tibial nerve behind the knee, or the brachial plexus at Erb's point. Furthermore, conduction block in nerve roots may not be apparent due to it not being possible to reliably stimulate them. In early Guillain-Barré or multi-focal motor neuropathy with conduction block this is relevant. In these cases conduction in the distal nerve segments may be normal. In inherited demyelinating neuropathies the myelin is affected in a uniform manner, and so while conduction velocity is reduced, there is generally no temporal dispersion or conduction block.[1]
- F-waves
In the median motor response figure, there is a supra-maximal stimulus to the median nerve at the wrist. The cathode is below the orange spot. A pair of action potentials in each of the motor fibres travel in opposite directions away from the cathode. The CAMP response is from action potentials traveling orthodromically. The antidromic action potentials travel towards the anterior horn cells. This elicits a further action potential in some of the anterior horn cells, which then travels back down the motor fibre, past the stimulating electrode, and activates the individual motor units that are supplied by those fibres. These action potentials that come much later are called the F-wave. F stands for foot, because they were first recorded in the foot. F-waves are variable. They may occur in a fast conducting fibre, a slower one, or not at all. The neurophysiologist must sample many responses (around 20), look at the proportion where there is an F-wave, and the range of latencies that are produced. [1]
The most important measurement for the F-wave is the minimum latency. The minimum latency represents conduction of the fastest fibres. The F-wave samples almost the entire nerve in both directions, and so it can pick up subtle changes that may not be present on standard motor conduction studies. But they have limited localising value. They are also the only way of looking at the most proximal nerve segment. In early Guillain-Barré syndrome the only finding may be an increased F-wave latency. A difference in latency of the ulnar F-wave between two sides can reflect a mild demyelinating lesion on the slower side, which also may not be apparent on motor conduction studies.[1]
- A-waves
Branches in motor axons cause A-waves. With F-wave testing, the antidromic potential travels up the nerve and it can take a "short-cut" back down to the muscle. Therefore, A-waves generally have a shorter latency than the F-wave. Their latency is static because they travel along the same fibres with each stimulus. A-waves can occur where collateral axonal sprouting is present, such as motor neuron disease, chronic focal neuropathies, and radiculopathies. However they have limited diagnostic value. The most important relevance of the A-wave is that they can be mistaken for F-waves, and if this occurs then the minimum F-wave latency value will be incorrect.[1]
- H reflex
This is a true reflex with an afferent arc that is mediated by large fast-conducting group 1a fibres, and an efferent arc that is mediated by alpha motor neurons. The H reflex is only visible with submaximal stimulation. It is seen most consistently with tibial nerve stimulation in the popliteal fossa with recording from the soleus and plantar foot muscles. The H reflex is useful for assessing the S1 nerve root in a suspected radiculopathy, and for assessing proximal conduction in polyneuropathies. [2]
Anatomical Variations
The most important anatomical variation to know about is the Martin-Gruber anastomosis. In this situation fibres pass from the median nerve to the ulnar nerve in the forearm, and they then innervate intrinsic hand muscles that are normally innervated by the ulnar nerve. With this anastomosis, there is a smaller amplitude with stimulation of the median nerve at the wrist compared to stimulation at the elbow; conversely, stimulation of the ulnar nerve at the elbow results in a smaller CMAP response compared to stimulation at the wrist. This NCS data can be incorrectly interpreted as there being a conduction block in the ulnar nerve, and the patient could end up having unnecessary decompression surgery. Other rarer variations to be aware of are an ulnar to median anastomosis in the forearm, and an accessory deep peroneal nerve.[1]
Key Points
- NCSs are a sensitive (but not specific) test for many peripheral nervous system diseases. Precise lesion localisation is possible.
- Essential measurements are SNAP and CMAP amplitude, area, duration, configuration, and conduction velocities.
- NCSs should be interpreted alongside electromyography.
- NCSs are generally normal in polyneuropathies with predominant small fibre neuropathy.
- NCSs are a snapshot in time, and may be normal during very early stages of an acquired inflammatory demyelinating neuropathy.
- The three main disease mechanisms that affect peripheral nerve are axonal degeneration, demyelination, and conduction block.
- Axonal degeneration: Reduced amplitude of SNAPs or CMAPs.
- Demyelination: Reduced conduction velocity.
- Conduction block: Acquired demyelinating nerve lesions. Looking at the amount of drop of CMAP amplitude and/or the area when stimulating from more proximal compared to distal sites.
- F-waves are useful for evaluating peripheral neuropathies with predominant proximal involvement.
See Also
References
- ↑ 1.00 1.01 1.02 1.03 1.04 1.05 1.06 1.07 1.08 1.09 1.10 1.11 1.12 1.13 1.14 1.15 1.16 1.17 1.18 1.19 1.20 Whittaker. SNAPs, CMAPs and F-waves: nerve conduction studies for the uninitiated. Practical neurology 2012. 12:108-15. PMID: 22450459. DOI.
- ↑ 2.00 2.01 2.02 2.03 2.04 2.05 2.06 2.07 2.08 2.09 2.10 2.11 2.12 2.13 2.14 2.15 2.16 2.17 2.18 Robert Henderson. Overview of nerve conduction studies. In: UpToDate, Post, TW (Ed), UpToDate, Waltham, MA, 2021.
- ↑ 3.0 3.1 Biller, José. Practical neurology. Philadelphia: Wolters Kluwer, 2017.
Literature Review
- Reviews from the last 7 years: review articles, free review articles, systematic reviews, meta-analyses, NCBI Bookshelf
- Articles from all years: PubMed search, Google Scholar search.
- TRIP Database: clinical publications about evidence-based medicine.
- Other Wikis: Radiopaedia, Wikipedia Search, Wikipedia I Feel Lucky, Orthobullets,